A Review of Mexican Contributions to Li₂CuO₂ and its Chemical Modifications as Cathode Materials for Lithium-Ion Batteries
DOI:
https://doi.org/10.29356/jmcs.v68i4.2294Keywords:
Li2CuO2, cation doping, anion doping, dual doping, in situ analysisAbstract
Over the past few decades, battery research has primarily focused on reducing costs and increasing energy density. There have been significant efforts to identify alternative cathode materials that could replace cobalt-based ones, with the goal of finding more environmentally friendly and cost-effective options. In this context, copper-based cathodes have emerged as promising candidates. The appeal of copper-based cathodes lies in their relatively high abundance, particularly in Mexico, their high theoretical energy density, and the potential to enhance their properties by altering their chemical structure. In recent years, numerous research initiatives in Mexico have aimed to make Li2CuO2 cathodes a viable option. This review examines the recent advances and future perspectives of these efforts, with a particular emphasis on the latest attempts to modify the synthesis route and incorporate multiple dopants to create synergistic effects.
Resumen. Durante las últimas décadas, la investigación sobre baterías se ha enfocado principalmente en la disminución de costos y el incremento de la densidad energética. Se han realizado importantes esfuerzos para identificar materiales catódicos alternativos que podrían reemplazar a los materiales basados en cobalto, con el objetivo de encontrar opciones rentables y con menor impacto al medio ambiente. En este contexto, los materiales catódicos basados en cobre se han convertido en candidatos prometedores. El interés por los cátodos basados en cobre radica en su abundancia relativamente alta, particularmente en México, su alta densidad energética teórica y la cualidad de mejorar sus propiedades alterando su estructura química. En los últimos años, numerosas propuestas de investigación en México han tenido como objetivo hacer de los cátodos de Li2CuO2 una opción viable. Este resumen recopila los avances recientes y las perspectivas a futuro de estos esfuerzos, con especial énfasis en los últimos intentos de modificar la ruta de síntesis y, a su vez, incorporar múltiples dopantes para crear efectos sinérgicos.
Downloads
References
Jäger-Waldau A. EPJ Photovoltaics, 2022, 13. DOI: https://doi.org/10.1051/epjpv/2022010.
Summerfield-Ryan O., Park S., Ecological Economics, 2023, 210, 107841. DOI: https://doi.org/10.1016/j.ecolecon.2023.107841.
Perez M., Perez R., Solar Energy Advances, 2022, 2, 100014. DOI: https://doi.org/10.1016/j.seja.2022.100014.
Vivoda V., Bazilian M.D., Khadim A., Ralph N., Krame G., Energy Res Soc Sci, 2024, 108, 103393. DOI: https://doi.org/10.1016/j.erss.2023.103393.
Hoppe R., Rieck H, Zeitschrift für anorganische und allgemeine Chemie, 1970, 379, 2, 157-164. DOI: https://doi.org/10.1002/zaac.19703790206.
Hoffmann R., Hoppe R., Schäfer W., Zeitschrift für anorganische und allgemeine Chemie, 1989, 578, 1, 18-26. DOI: https://doi.org/10.1002/zaac.19895780103.
Sapiña F., Rodríguez-Carvajal J., Sanchis M.J., Ibáñez R., Beltrán A., Beltrán D., Solid State Commun, 1990, 74, 779–784. DOI: https://doi.org/10.1016/0038-1098(90)90934-4.
Prakash A.S., Larcher D., Morcrette M., Hegde M.S., Leriche J., 2005, 74, 4406–4415. DOI: https://doi.org/10.1021/cm0508266.
Ruther R.E., Samuthira Pandian A., Yan P., Weker J.N., Wang C., Nanda J., Chemistry of Materials, 2017, 29, 2997–3005. DOI: https://doi.org/10.1021/acs.chemmater.6b05442.
Ruther R.E., Zhou H., Dhital C., Saravanan K., Kercher A.K., Chen G., et al., Chemistry of Materials, 2015, 27, 6746–6754. DOI: https://doi.org/10.1021/acs.chemmater.5b02843.
Xu J., Renfrew S., Marcus M.A., Sun M., McCloskey B.D., Tong W., Journal of Physical Chemistry C, 2017, 121, 11100–11107. DOI: https://doi.org/10.1021/acs.jpcc.7b01799.
Martínez-Cruz M.A., Yañez-Aulestia A., Ramos-Sánchez G., Oliver-Tolentino M., Vera M., Pfeiffer H., et al., Dalton Transactions, 2020, 49, 4549–4558. DOI: https://doi.org/10.1039/D0DT00273A.
Arachi Y., Ide T., Nakagawa T., Nakata Y., ECS Trans, 2012, 50, 143–151. DOI: https://doi.org/10.1149/05024.0143ecst.
Perea-Ramírez L.I., Guevara-García A., Galván M., J Mol Model, 2018, 24, 227. DOI: https://doi.org/10.1007/s00894-018-3754-0.
Juarez-Yescas C., Oliver-Tolentino M., Ramos-Sánchez G., Vera-Ramirez M.A., Olmedo-González J., Ochoa-Calle A., et al., ACS Appl Energy Mater, 2020, 3, 2771–2780. DOI: https://doi.org/10.1021/acsaem.9b02429.
Ramos-Sanchez G., Romero-Ibarra I.C., Vazquez-Arenas J., Tapia C., Aguilar-Eseiza N., Gonzalez I., Solid State Ion, 2017, 303, 89–96. DOI: https://doi.org/10.1016/j.ssi.2017.02.018.
Aguilar-Eseiza N., Ramos-Sánchez G., González F., González I., Electrochem Commun, 2018, 96, 32–36. DOI: https://doi.org/10.1016/j.elecom.2018.09.002.
Shannon R.D., Acta Crystallographica Section A, 1976, 32, 768–771. DOI: https://doi.org/10.1107/S0567739476001551.
Mizuno Y., Tohyama T., Maekawa S., Phys Rev B Condens Matter Mater Phys, 1999, 60, 6230–6233. DOI: https://doi.org/10.1103/PhysRevB.60.6230.
Garcia Carrillo B.A., Estudio de cuprato de litio modificado con iones de metales de transición como cátodo alternativo para baterías de ion litio, Universidad Autónoma Metropolitana, 2023
Weng Y., Zhang H., Ionics (Kiel), 2024, 30, 1885–1895. DOI: https://doi.org/10.1007/s11581-023-05366-4.
He Z., Zhang M., Zhou K., Cheng Y., Luo M., Su Y., et al., ACS Appl Energy Mater, 2023, 6, 3422–3431. DOI: https://doi.org/10.1021/acsaem.2c04133.
Ahn H., Choi J., Kim M., Kyu Kang S., Jang D., Maeng J., et al., Chemistry of Materials, 2024, 36, 9, 4379-4392. DOI: https://doi.org/10.1021/acs.chemmater.3c03307.
Kong F., Liang C., Longo R.C., Yeon D.H., Zheng Y., Park J.H., et al., Chemistry of Materials, 2016, 28, 6942–6952. DOI: https://doi.org/10.1021/acs.chemmater.6b02627.
Kim H., Kim S.B., Park D.H., Park K.W., Energies (Basel), 2020, 13, 4808-4817. DOI: https://doi.org/10.3390/en13184808.
Lin Y., Zhong K., Zheng J., Liang M., Xu G., Feng Q., et al., ACS Appl Energy Mater, 2021, 4, 9848–9857. DOI: https://doi.org/10.1021/acsaem.1c01883.
Dai K., Wu J., Zhuo Z., Li Q., Sallis S., Mao J., et al., Joule, 2019, 3, 518–541. DOI: https://doi.org/10.1016/j.joule.2018.11.014.
Martínez-Cruz M.A., Ramos-Sánchez G., Oliver-Tolentino M., Pfeiffer H., González I., J Alloys Compd, 2022, 923, 166328. DOI: https://doi.org/10.1016/j.jallcom.2022.166328.
Zhang Y., Li H., Liu J., Liu J., Ma H., Cheng F., Journal of Energy Chemistry, 2021, 63, 312-319. DOI: https://doi.org/10.1016/j.jechem.2021.07.029.
Ryu H.H., Park G.T., Yoon C.S., Sun Y.K., J Mater Chem A Mater, 2019, 7, 18580–18588. DOI: https://doi.org/10.1039/C9TA06402H.
Hao Q., Du F., Xu T., Zhou Q., Cao H., Fan Z., et al., Journal of Electroanalytical Chemistry, 2022, 907, 116034. DOI: https://doi.org/10.1016/j.jelechem.2022.116034.
Goonetilleke D., Mazilkin A., Weber D., Ma Y., Fauth F., Janek J., et al., J Mater Chem A Mater, 2022, 10, 7841–7855. DOI: https://doi.org/10.1039/D1TA10568J.
Zhang F., Zhou X., Fu X., Wang C., Wang B., Liang W., et al., Mater Today Energy, 2021, 22, 100873. DOI: https://doi.org/10.1016/j.mtener.2021.100873.
Li J., Cameron A.R., Li H., Glazier S., Xiong D., Chatzidakis M., et al., J Electrochem Soc, 2017, 164, A1534–A1544. DOI: https://doi.org/10.1149/2.0991707jes.
Balodhi A., Kim M.G., Crystals (Basel), 2024, 14, 288. DOI: https://doi.org/10.3390/cryst14030288.
Kawamata S., Okuda K., Kindo K., J Magn Magn Mater, 2004, 272–276, 939–940. DOI: https://doi.org/10.1016/j.jmmm.2003.12.579.
Hausbrand R., Cherkashinin G., Ehrenberg H., Gröting M., Albe K., Hess C., et al., Materials Science and Engineering: B, 2015, 192, 3–25. DOI: https://doi.org/10.1016/j.mseb.2014.11.014.
Minato T., Abe T., Prog Surf Sci, 2017, 92, 240–280. DOI: https://doi.org/10.1016/j.progsurf.2017.10.001.
Xin F., Zhou H., Chen X., Zuba M., Chernova N., Zhou G., et al., ACS Appl Mater Interfaces, 2019, 11, 34889–34894. DOI: https://doi.org/10.1021/acsami.9b09696.
Takada K., Ohta N., Zhang L., Fukuda K., Sakaguchi I., Ma R., et al., Solid State Ion, 2008, 179, 1333–1337. DOI: https://doi.org/10.1016/j.ssi.2008.02.017.
Li X., Jin L., Song D., Zhang H., Shi X., Wang Z., et al., Journal of Energy Chemistry, 2020, 40, 39–45. DOI: https://doi.org/10.1016/j.jechem.2019.02.006.
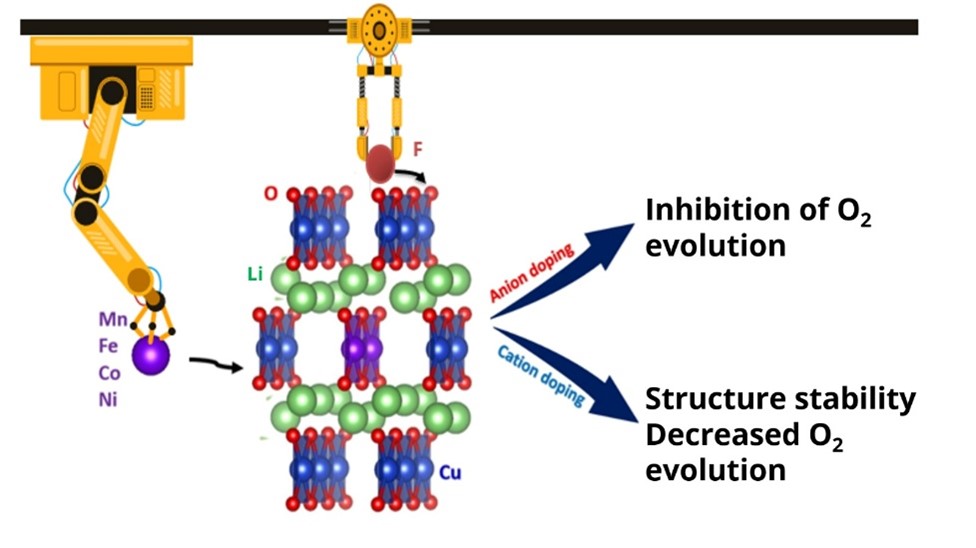
Downloads
Published
Issue
Section
License
Copyright (c) 2024 B.A. García-Carrillo, A de J Martínez, E. L. Jiménez-Cabañas, MA Martínez-Cruz, C. Juárez-Yescas, G. Ramos-Sánchez

This work is licensed under a Creative Commons Attribution-NonCommercial 4.0 International License.
Authors who publish with this journal agree to the following terms:
- Authors retain copyright and grant the journal right of first publication with the work simultaneously licensed under a Creative Commons Attribution License that allows others to share the work with an acknowledgement of the work's authorship and initial publication in this journal.
- Authors are able to enter into separate, additional contractual arrangements for the non-exclusive distribution of the journal's published version of the work (e.g., post it to an institutional repository or publish it in a book), with an acknowledgement of its initial publication in this journal.
