The Use of Grand Canonical Density Functional Theory Global and Local Reactivity Parameters to Study Electrochemical Processes in Energy Storage Materials
DOI:
https://doi.org/10.29356/jmcs.v69i1.2289Keywords:
Grand canonical density functional theory, solvent-free reactions, quantum capacitance, oxygen evolutionAbstract
In this review a summary of the results obtained in a collaboration between electrochemists and theoretical physical chemists in the chemistry department of the Metropolitan Autonomous University Campus Iztapalapa in recent years is presented. The focus is on the study of materials involved in electrochemical storage devices such as batteries and capacitors, and in advance electrochemical oxidation reactions. Along this collaboration, the combination of density functional theory reactivity parameters within the Grand Canonical Density Functional Theory give rise to an alternative approach to follow redox processes in bulk materials and in solid-liquid interfaces. Along the review, we show how global and local softness emerge as suitable and ad hoc quantities to analyze electrochemical experiments. The use of local softness to distinguish “innocent” coordination environments from the active ones is exemplified with representative systems. A robust method to estimate quantum and total capacitances is described in the case of substituted graphene and is also applicable to other systems. Also, the introduction of an absolute approximate scale to characterize the redox properties of electrode-solvent systems is illustrated for a set of well-known surfaces of transition metal oxides.
Resumen. En esta revisión se presenta un resumen de los resultados obtenidos de una colaboración entre electroquímicos y fiscoquímicos teóricos del departamento de química de la Universidad Autónoma Metropolitana Unidad Iztapalapa en los últimos años. Se hace énfasis en el estudio de los materiales implicados en los dispositivos de almacenamiento electroquímico de energía, como baterías y capacitores, y en las reacciones de oxidación electroquímica avanzada. Se analiza el uso de los parámetros de reactividad definidos en la Teoría de Funcionales de la Densidad en su formulación Gran Canónica para estudiar procesos redox en el bulto de los materiales y en intercaras sólido-líquido. Se muestran ejemplos de cómo la blandura química global y local emergen como cantidades ad hoc para analizar el comportamiento de los materiales que participan en procesos electroquímicos. En partícular, se muestra un ejemplo del uso de la blandura química local para distinguir, en procesos redox, los entornos de coordinación "inocentes" de los activos. Se describe también un método para estimar las capacitancias cuánticas y totales en el caso del grafeno sustituido y que es aplicable a intercaras sólido-liquido en general. Por otra parte, se ilustra, para un conjunto de superficies bien conocidas de óxidos de metales de transición, el uso de una escala absoluta aproximada para caracterizar en forma relativa las propiedades redox de los sistemas electrodo-disolvente.
Downloads
References
Tavernelli, I.; Vuilleumier, R.; Sprik, M. Phys. Rev. Lett. 2002, 88, 213002. DOI: https://doi.org/10.1103/PhysRevLett.88.213002.
Tateyama, Y.; Blumberger, J.; Sprik, M.; Tavernelli, I. J. Chem. Phys. 2005, 122, 234505. DOI: https://doi.org/10.1063/1.1938192.
Sundararaman, R.; Vigil-Fowler, D.; Schwarz, K. Chem. Rev. 2022, 122, 10651-10674. DOI: https://doi.org/10.1021/acs.chemrev.1c00800.
Saubanère, M.; Yahia, M. B.; Lebègue, S.; Doublet, M. L. Nature 2014, 5, 5559. DOI: https://doi.org/10.1038/ncomms6559.
Neugebauer, H.; Bohle, F.; Bursch, M.; Hansen, A.; Grimme, S. J. Phys. Chem. A. 2020, 124, 7166-7176. DOI: https://doi.org/10.1021/acs.jpca.0c05052.
Meng, Y. S.; Arroyo-de Dompablo, M. E. Energ. Environ. Sci. 2009, 2, 589-609. DOI: https://doi.org/10.1039/b901825e.
Li, M.; Yang, X.; Xue, Y. Theor. Chem. Acc. 2017, 136, 69. DOI: https://doi.org/10.1007/s00214-017-2103-1.
Jaque, P.; Marenich, A. V.; Cramer, C. J.; Truhlar, D. G. J. Phys. Chem. C. 2007, 111, 5783-5799. DOI: https://doi.org/10.1021/jp066765w.
Fornari, R. P.; de Silva, P. Molecules. 2021, 26, 3978. DOI: https://doi.org/10.3390/molecules26133978.
Fedorov, R.; Gryn’ova, G. J. Chem. Theor. Comp. 2023, 19, 4796-4814. DOI: https://doi.org/10.1021/acs.jctc.3c00355.
Cramer, C. J.; Truhlar, D. G. Phys. Chem. Chem. Phys. 2009, 11, 10757-10816. DOI: https://doi.org/10.1039/b907148b.
Bhattacharjee, S.; Isegawa, M.; Garcia-Ratés, M.; Neese, F.; Pantazis, D. A. J. Chem. Theor. Comp. 2022, 18, 1619-1632. DOI: https://doi.org/10.1021/acs.jctc.1c01267.
Arumugam, K.; Becker, U. Minerals. 2014, 4, 345-387. DOI: https://doi.org/10.3390/min4020345.
Cheng, J.; Sulpizi, M.; Sprik, M. J. Chem. Phys. 2009, 131, 154504. DOI: https://doi.org/10.1063/1.3250438.
Blumberger, J.; Bernasconi, L.; Tavernelli, I.; Vuilleumier, R.; Sprik, M. J. Am. Chem. Soc. 2004, 126, 3928-3938. DOI: https://doi.org/10.1021/ja0390754.
Moens, J.; Jaque, P.; De Proft, F.; Geerlings, P. J. Phys. Chem. A. 2008, 112, 6023-6031. DOI: https://doi.org/10.1021/jp711652a.
Moens, J.; Geerlings, P.; Roos, G. Chemistry. 2007, 13, 8174-84. DOI: https://doi.org/10.1002/chem.200601896.
Miranda-Quintana, R. A.; Martinez Gonzalez, M.; Ayers, P. W. Phys. Chem. Chem. Phys. 2016, 18, 22235-22243. DOI: https://doi.org/10.1039/c6cp03213c.
Miranda, D. A.; Bueno, P. R. Phys. Chem. Chem. Phys. 2016, 18, 25984-25992. DOI: https://doi.org/10.1039/c6cp01659f.
Filhol, J. S.; Doublet, M. L. J. Phys. Chem. C. 2014, 118, 19023-19031. DOI: https://doi.org/10.1021/jp502296p.
Feliciano, G. T.; Bueno, P. R. J. Phys. Chem. C. 2020, 124, 14918-14927. DOI: https://doi.org/10.1021/acs.jpcc.0c04598.
Bueno, P. R.; Miranda, D. A. Phys. Chem. Chem. Phys. 2017, 19, 6184-6195. DOI: https://doi.org/10.1039/c6cp02504h.
Bueno, P. R.; Feliciano, G. T.; Davis, J. J. Phys. Chem. Chem. Phys. 2015, 17, 9375-9382. DOI: https://doi.org/10.1039/c4cp06015f.
Zheng, Y.; Jiao, Y.; Jaroniec, M.; Qiao, S. Z. Angew. Chem. Int. Ed. 2015, 54, 52-65. DOI: https://doi.org/10.1002/anie.201407031.
Parr, R. G.; Yang, W. Density-Functional Theory of Atoms and Molecules. New York: Oxford University Press, 1989.
Geerlings, P.; De Proft, F.; Langenaeker, W. Chem. Rev. 2003, 103, 1793-1874. DOI: https://doi.org/10.1021/cr990029p.
Geerlings, P.; Chamorro, E.; Chattaraj, P. K.; De Proft, F.; Gázquez, J. L.; Liu, S.; Morell, C.; Toro-Labbé, A.; Vela, A.; Ayers, P. Theor. Chem. Acc. 2020, 139, 36. DOI: https://doi.org/10.1007/s00214-020-2546-7.
Gázquez, J. L.; Franco-Pérez, M.; Ayers, P. W.; Vela, A. Int. J. Quantum Chem. 2019, 119, e25797. DOI: https://doi.org/https://doi.org/10.1002/qua.25797.
Kohn, W.; Vashishta, P., in: Theory of the Inhomogeneous Electron gas, Lundqvist, S. and March, N. H. Eds., Plenum, New York, 1983.
Mermin, N. D. Phys. Rev. 1965, 137, A1441. DOI: https://doi.org/10.1103/PhysRev.137.A1441.
Sundararaman, R.; Schwarz, K. A.; Letchworth-Weaver, K.; Arias, T. A. J. Chem. Phys. 2015, 142, 054102. DOI: https://doi.org/10.1063/1.4906828.
Sundararaman, R.; Letchworth-Weaver, K.; Schwarz, K. A. J. Chem. Phys. 2018, 148, 144105. DOI: https://doi.org/10.1063/1.5024219.
Sundararaman, R.; Letchworth-Weaver, K.; Arias, T. A. J. Chem. Phys. 2014, 140, 144504. DOI: https://doi.org/10.1063/1.4870653.
Sundararaman, R.; Arias, T. A. Comput. Phys. Commun. 2014, 185, 818-825. DOI: https://doi.org/10.1016/j.cpc.2013.11.013.
Petrosyan, S. A.; Briere, J.-F.; Roundy, D.; Arias, T. A. Phys. Rev. B. 2007, 75, 205105. DOI: https://doi.org/10.1103/PhysRevB.75.205105.
Mathew, K.; Sundararaman, R.; Letchworth-Weaver, K.; Arias, T. A.; Hennig, R. G. J. Chem. Phys. 2014, 140, 084106. DOI: https://doi.org/10.1063/1.4865107.
Letchworth-Weaver, K.; Arias, T. A. Phys. Rev. B. 2012, 86, 075140. DOI: https://doi.org/10.1103/PhysRevB.86.075140.
Gunceler, D.; Letchworth-Weaver, K.; Sundararaman, R.; Schwarz, K. A.; Arias, T. A. Modell. Simul. Mater. Sci. Eng. 2013, 21, 074005. DOI: https://doi.org/10.1088/0965-0393/21/7/074005.
Franco-Pérez, M.; Gázquez, J. L.; Ayers, P. W.; Vela, A. J. Chem. Phys. 2015, 143, 154103. DOI: https://doi.org/10.1063/1.4932539.
Yang, W.; Parr, R. G. Proc. Natl. Acad. Sci. U.S.A. 1985, 82, 6723-6726. DOI: https://doi.org/10.1073/pnas.82.20.6723.
Vela, A.; Gazquez, J. L. J. Am. Chem. Soc. 1990, 112, 1490-1492. DOI: https://doi.org/10.1021/ja00160a029.
Parr, R. G.; Yang, W. J. Am. Chem. Soc. 1984, 106, 4049-4050. DOI: https://doi.org/10.1021/ja00326a036.
Goodenough, J. B. Solid State Ionics. 1994, 69, 184-198. DOI: https://doi.org/10.1016/0167-2738(94)90409-X.
Armand, M. B., in: Materials for Advanced Batteries, Murphy, D. W., Broadhead, J., and Steele, B. C. H. Eds., Springer, 1980, 145-161.
Kim, T.; Song, W.; Son, D.-Y.; Ono, L. K.; Qi, Y. J. Mater. Chem. A. 2019, 7, 2942-2964. DOI: https://doi.org/10.1039/C8TA10513H.
Islas-Vargas, C.; Guevara-García, A.; Oliver-Tolentino, M.; Ramos-Sánchez, G.; González, I.; Galván, M. J. Electrochem. Soc. 2019, 166, A5139. DOI: https://doi.org/10.1149/2.0231903jes.
Johannes, M.; Swider-Lyons, K.; Love, C. T. Solid State Ionics. 2016, 286, 83-89. DOI: https://doi.org/10.1016/j.ssi.2015.12.025.
Johannes, M.; Hoang, K.; Allen, J.; Gaskell, K. Phys. Rev. B. 2012, 85, 115106. DOI: https://doi.org/10.1103/PhysRevB.85.115106.
Perea-Ramírez, L. I.; Guevara-García, A.; Galván, M. J. Mol. Model. 2018, 24, 227. DOI: https://doi.org/10.1007/s00894-018-3754-0.
Cohen, M. H.; Ganduglia‐Pirovano, M. V.; Kudrnovský, J. J. Chem. Phys. 1994, 101, 8988-8997. DOI: https://doi.org/10.1063/1.468026.
Hasan, M. H.; McCrum, I. T. Curr. Opin. Electrochem. 2022, 33, 100937. DOI: https://doi.org/10.1016/j.coelec.2022.100937.
Zhang, Y.; Cummings, P. T. ACS Appl. Mater. Interfaces. 2019, 11, 42680-42689. DOI: https://doi.org/10.1021/acsami.9b09939.
Sundararaman, R.; Goddard, W. A.; Arias, T. A. J. Chem. Phys. 2017, 146, 114104. DOI: https://doi.org/10.1063/1.4978411.
Tomasi, J.; Mennucci, B.; Cammi, R. Chem. Rev. 2005, 105, 2999-3094. DOI: https://doi.org/10.1021/cr9904009.
Klamt, A. Wiley Interdiscip. Rev. Comput. Mol. Sci. 2018, 8, e1338. DOI: https://doi.org/10.1002/wcms.1338.
Mennucci, B. Wiley Interdiscip. Rev. Comput. Mol. Sci. 2012, 2, 386-404. DOI: https://doi.org/10.1002/wcms.1086.
Cramer, C. J.; Truhlar, D. G. Chem. Rev. 1999, 99, 2161-2200. DOI: https://doi.org/10.1021/cr960149m.
Kohn, W.; Sham, L. J. Phys. Rev. 1965, 140, A1133. DOI: https://doi.org/10.1103/PhysRev.140.A1133.
Grimme, S. J. Comput. Chem. 2006, 27, 1787-1799. DOI: https://doi.org/10.1002/jcc.20495.
Sundararaman, R.; Goddard, W. A., 3rd. J. Chem. Phys. 2015, 142, 064107. DOI: https://doi.org/10.1063/1.4907731.
Luryi, S. Appl. Phys. Lett. 1988, 52, 501-503. DOI: https://doi.org/10.1063/1.99649.
Paek, E.; Pak, A. J.; Hwang, G. S. J. Electrochem. Soc. 2013, 160, A1. DOI: https://doi.org/10.1149/2.019301jes.
Zhan, C.; Jiang, D.-e. J. Phys. Chem. Lett. 2016, 7, 789-794. DOI: https://doi.org/10.1021/acs.jpclett.6b00047.
Bard, A. J.; Faulkner, L. R. Electrochemical Methods: Fundamentals and Applications. Wiley, 2012.
Bo, Z.; Li, C.; Yang, H.; Ostrikov, K.; Yan, J.; Cen, K. Nano-Micro Lett. 2018, 10, 33. DOI: https://doi.org/10.1007/s40820-018-0188-2.
Górniak, R.; Lamperski, S. J. Phys. Chem. C. 2014, 118, 3156-3161. DOI: https://doi.org/10.1021/jp411698w.
Perdew, J. P. Phys. Rev. B. 1988, 37, 6175. DOI: https://doi.org/10.1103/physrevb.37.6175.
Sabin, J. R.; Trickey, S.; Apell, S. P.; Oddershede, J. Int. J. Quantum Chem. 2000, 77, 358-366. DOI: https://doi.org/10.1002/(SICI)1097-461X(2000)77:1<358::AID-QUA35>3.0.CO;2-D.
Iafrate, G.; Hess, K.; Krieger, J.; Macucci, M. Phys. Rev. B. 1995, 52, 10737. DOI: https://doi.org/10.1103/physrevb.52.10737.
Cohen, M. H.; Ganduglia-Pirovano, M. V.; Kudrnovský, J. Phys. Rev. Lett. 1994, 72, 3222-3225. DOI: https://doi.org/10.1103/PhysRevLett.72.3222.
Cohen, M.; Ganduglia‐Pirovano, M.; Kudrnovský, J. J. Chem. Phys. 1994, 101, 8988-8997. DOI: https://doi.org/10.1063/1.468026.
Cohen, M. H.; Ganduglia‐Pirovano, M. V.; Kudrnovský, J. J. Chem. Phys. 1995, 103, 3543-3551. DOI: https://doi.org/10.1063/1.470238.
Wilke, S.; Cohen, M. H.; Scheffler, M. Phys. Rev. Lett. 1996, 77, 1560-1563. DOI: https://doi.org/10.1103/PhysRevLett.77.1560.
Galvan, M.; Dal Pino Jr, A.; Wang, J.; Joannopoulos, J. D. J. Phys. Chem. 1993, 97, 783-785. DOI: https://doi.org/10.1021/j100106a001.
Szarek, P. J. Phys. Chem. C. 2016, 120, 17175-17183. DOI: https://doi.org/10.1021/acs.jpcc.6b03752.
Ochoa-Calle, A.; Guevara-García, A.; Vazquez-Arenas, J.; González, I.; Galván, M. J. Phys. Chem. A. 2020, 124, 573-581. DOI: https://doi.org/10.1021/acs.jpca.9b10885.
Pletcher, D., in: Electrochemistry for the environment, Comninellis, C. and Chen, G. Eds., Springer, 2010.
Comninellis, C. Electrochim. Acta. 1994, 39, 1857-1862. DOI: https://doi.org/10.1016/0013-4686(94)85175-1.
Jaimes, R.; Vazquez-Arenas, J.; González, I.; Galván, M. Electrochim. Acta. 2017, 229, 345-351. DOI: https://doi.org/10.1016/j.electacta.2017.01.120.
Jaimes, R.; Vazquez-Arenas, J.; González, I.; Galván, M. Surface Science. 2016, 653, 27-33. DOI: https://doi.org/10.1016/j.susc.2016.04.018.
Islas-Vargas, C.; Guevara-García, A.; Galván, M. J. Chem. Phys. 2021, 154, 074704. DOI: https://doi.org/10.1063/5.0035208.
Ping, Y.; Sundararaman, R.; Goddard III, W. A. Phys. Chem. Chem. Phys. 2015, 17, 30499-30509. DOI: https://doi.org/10.1039/c5cp05740j.
Islas-Vargas, C.; Guevara-García, A.; Galván, M. Theor. Chem. Acc. 2024, 143, 34. DOI: https://doi.org/10.1007/s00214-024-03103-2.
Marselli, B.; Garcia-Gomez, J.; Michaud, P.-A.; Rodrigo, M. A.; Comninellis, C. J. Electrochem. Soc. 2003, 150, D79. DOI: https://doi.org/10.1149/1.1553790.
Martinez-Huitle, C. A.; Ferro, S. Chem. Soc. Rev. 2006, 35, 1324-1340. DOI: https://doi.org/10.1039/b517632h.
Koppenol, W.; Liebman, J. F. J. Phys. Chem. 1984, 88, 99-101. DOI: https://doi.org/10.1021/j150645a024.
Berdinko, V.; Bazhin, N. Russ. J. Phys. Chem. 1970, 44, 395.
Li, A.; Weng, J.; Yan, X.; Li, H.; Shi, H.; Wu, X. J. Electroanal. Chem. 2021, 898, 115622. DOI: https://doi.org/10.1016/j.jelechem.2021.115622.
Smith, J. C.; Pribram-Jones, A.; Burke, K. Phys. Rev. B. 2016, 93, 245131. DOI: https://doi.org/10.1103/PhysRevB.93.245131.
Harding, B. P.; Mauri, Z.; Xie, V. W.; Pribram-Jones, A. J. Chem. Phys. 2024, 160, 154108. DOI: https://doi.org/10.1063/5.0196650.
Burke, K.; Smith, J. C.; Grabowski, P. E.; Pribram-Jones, A. Phys. Rev. B. 2016, 93, 195132. DOI: https://doi.org/10.1103/PhysRevB.93.195132.
Kozlowski, J.; Perchak, D.; Burke, K. arXiv preprint arXiv:2308.03319. 2023, DOI: https://doi.org/10.48550/arXiv.2308.03319.
Sundararaman, R.; Schwarz, K. J. Chem. Phys. 2017, 146, 084111. DOI: https://doi.org/10.1063/1.4976971.
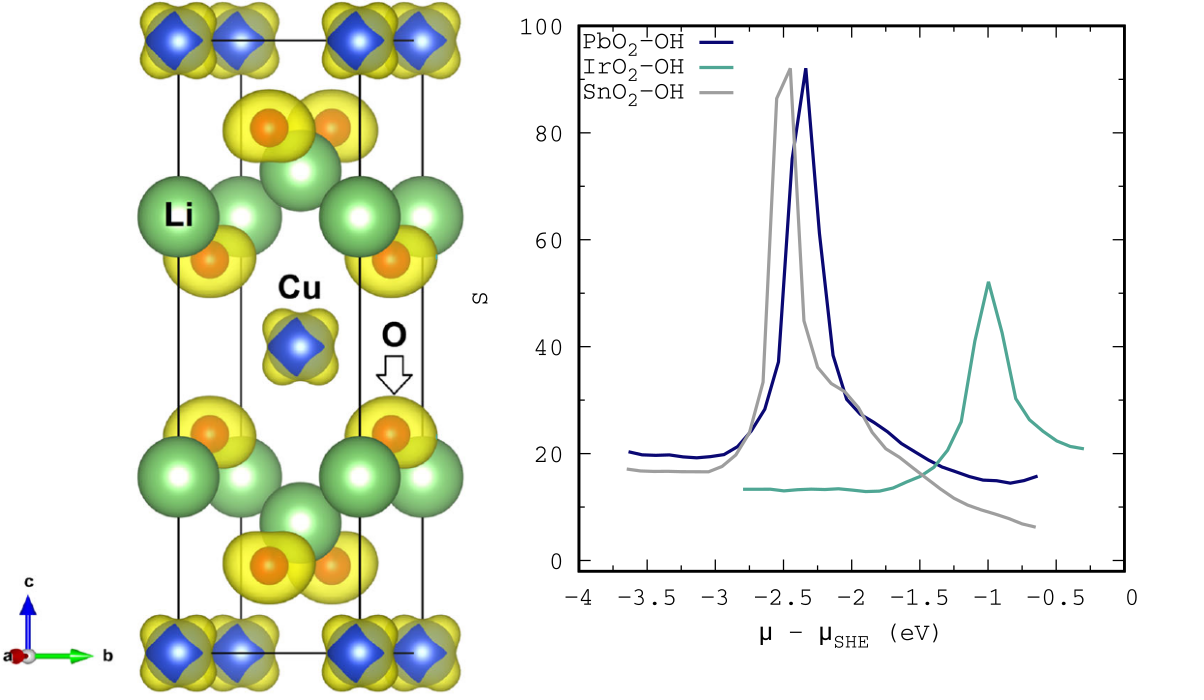
Downloads
Published
Issue
Section
License
Copyright (c) 2024 Claudia Islas-Vargas, Alfredo Guevara-García, Marcelo Galván

This work is licensed under a Creative Commons Attribution-NonCommercial 4.0 International License.
Authors who publish with this journal agree to the following terms:
- Authors retain copyright and grant the journal right of first publication with the work simultaneously licensed under a Creative Commons Attribution License that allows others to share the work with an acknowledgement of the work's authorship and initial publication in this journal.
- Authors are able to enter into separate, additional contractual arrangements for the non-exclusive distribution of the journal's published version of the work (e.g., post it to an institutional repository or publish it in a book), with an acknowledgement of its initial publication in this journal.
