Efficiency of Cattail to Remove a Mixture of Pharmaceuticals in a Constructed Wetland
DOI:
https://doi.org/10.29356/jmcs.v67i1.1848Keywords:
Constructed wetland, Typha latifolia, molecular interactions, pharmaceuticals, removal efficiencyAbstract
Reducing the risk of the aquatic ecosystem’s contamination with organic substances such as pharmaceuticals is of great importance due to the toxicological effect of these substances on aquatic organisms. Therefore, the removal of pharmaceuticals and/or their metabolites frequently reported in industrial or municipal wastewaters require efficient processes that complement the conventional treatment systems; the constructed wetlands are an option. In this work, the removal efficiency of mixed diclofenac and naproxen was evaluated using a subsurface flow constructed wetland planted with cattail (Typha latifolia), operated during 100 days at a hydraulic residence time (HRT) of 3 days. Under these conditions, the diclofenac and naproxen removal efficiencies were 82.0% and 74.5%, respectively. The interaction between diclofenac and naproxen with cellulose, which is the major cell wall compound of higher plants, was computationally modeled at the PM6 semi-empirical level of theory and it was found that diclofenac and naproxen interact with cellulose via hydrogen bonds.
Resumen. Reducir el riesgo de contaminación de los ecosistemas acuáticos con sustancias orgánicas como los fármacos es de gran importancia debido al efecto toxicológico de estas sustancias para los organismos acuáticos. Por ello, la remoción de los fármacos y/o sus metabolitos frecuentemente reportados en aguas residuales industriales o municipales requiere de procesos eficientes que complementen los sistemas convencionales de tratamiento; los humedales construidos son una opción. En este trabajo, se evaluó la eficiencia de remoción de diclofenaco y de naproxeno en mezcla utilizando un humedal construido de flujo subsuperficial con plantas de tule (Typha latifolia), operado durante 100 días con un tiempo de residencia hidráulica (TRH) de 3 días. En estas condiciones, las eficiencias de remoción de diclofenaco y de naproxeno fueron de 82.0 % y 74.5 % respectivamente. La interacción entre diclofenaco y naproxeno con celulosa, que es el componente mayoritario de la pared celular de las plantas superiores, se modeló computacionalmente al nivel de teoría semiempírico con el método PM6, y se encontró que diclofenaco y naproxeno interactúan con celulosa mediante puentes de hidrógeno.
Downloads
References
Parolini, M. Sci. Total Environ. 2020, 740, 140043. DOI: http://doi.org/10.1016/j.scitotenv.2020.140043
Bagnis, S.; Fitzsimons, M. F.; Snape, J.; Tappin, A.; Comber, S. Environ. Chem. Lett. 2018, 16, 1193–1216. DOI: http://doi.org/10.1007/s10311-018-0742-7
Verbruggen, B.; Gunnarsson, L.; Kristiansson, E.; Österlund, T.; Owen, S. F.; Snape, J. R.; Tyler, C. R. Nucleic Acids Res. 2017, 46, D930—D936. DOI: https://doi.org/10.1093/nar/gkx1024
Beek, T.; Weber, F.-A.; Bergmann, A.; Hickmann, S.; Ebert, I.; Hein, A.; Küster, A. Environ. Toxicol. Chem. 2016, 35, 823-835.
Peña-Álvarez, A.; Castillo-Alanís, A. TIP. 2015, 18, 29–42. DOI: https://doi.org/10.1016/j.recqb.2015.05.003
Rivera-Jaimes, J. A.; Postigo, C.; Melgoza-Alemán, R. M.; Aceña, J.; Barceló, D.; López de Alda, M. Sci. Total Environ. 2018, 613-614, 1263–1274. DOI: https://doi.org/10.1016/j.scitotenv.2017.09.134
Brodin, T.; Piovano, S.; Fick, J.; Klaminder, J.; Heynen, M.; Jonsson, M. Philos. Trans. R. Soc., B. 2014, 369, 1656. DOI: https://doi.org/10.1098/rstb.2013.0580
Brozinski, J.-M.; Lahti, M.; Meierjohann, A.; Oikari, A.; Kronberg, L. Environ. Sci. Technol. 2013, 47, 342–348. DOI: https://doi.org/10.1021/es303013j
Yang, Y.; Ok, Y. S.; Kim, K.-H.; Kwon, E. E.; Tsang, Y. F. Sci. Total Environ. 2017, 596-597, 303–320. DOI: https://10.1016/j.scitotenv.2017.04.102
Vystavna, Y.; Frkova, Z.; Marchand, L.; Vergeles, Y.; Stolberg, F. Ecological Engineering 2017, 108, 50-58.
Domaradzka, D.; Guzik, U.; Wojcieszyńska, D. Rev. Environ. Sci. Bio/Technol. 2015, 14, 229 –239. DOI: https://doi.org/10.1007/s11157-015-9364-8
Bayati, M; Ho, T.L.; Vu, D.C., Wang, F.; Rogers, E.; Cuvellier, C.; Huebotter, S.; Inniss, E.C.; Udawatta, R.; Jose, S.; Lin, C.-H. Int. J. Hyg. Environ. Health 2021, 231, 113664. DOI: https://doi.org/10.1016/j.ijheh.2020.113664
Cuevas, G.; Cortés, F. Introducción a la química computacional, Ed. Fondo de Cultura Económica, 2003.
Taylor, N.G. New Phytol. 2008, 178, 239-252. DOI: https://doi.org/10.1111/j.1469-8137.2008.02385.x
Reed, S. C. WWPCDM74. United States Environmental Protection Agency, USA. 1993.
Loredo, P.R.; Leura, V.A.K.; Monreal, M. A.; Mitchell, K. N.; Alfaro, M.C.; García, R. F. 11th International Conference on the Biogeochemistry of Trace elements. 2011.
Zapata-Morales, A. L.; Alfaro-De la Torre, M. C.; Hernández-Morales, A.; García-De la Cruz, R. F. Water, Air, Soil Pollut. 2020, 231, 423. DOI: https://doi.org/10.1007/s11270-020-04781-x
Barry, A.; Mahood, M.; Hamezh, M. J. Journal of Kerbala University 2009, 7, 310–316.
Alizadeh, N.; Keyhanian, F. Acta Poloniae Pharmaceutica - Drug Research 2015, 72, 867–875.
Arnon, D. Plant Physiol. 1949, 24, 1-15.
Stewart, J. J. P. J. Comput. Chem. 1989, 10, 209–220. DOI: https://doi.org/10.1002/jcc.540100208
Frisch, M.J.; Trucks, G.; Schlegel, H.B.; Scuseria, G.E.; Robb, M.A.; Cheeseman, J.; Scalmani, G.; Barone, V.; Mennucci, B.; Petersson, G.A.; Nakatsuji, H.; Caricato, M.; Li, X.; Hratchian, H.P.; Izmaylov, A.F.; Bloino, J.; Zheng, G.; Sonnenberg, J.; Hada, M.; Fox, D. Gaussian 09 Revision A.1. Gaussian Inc., Wallingford, CT, 2009.
Jalbout, A. F. Acta Chim. Slov. 2003, 50, 159-160.
Jensen, F. Introduction to Computational Chemistry. 3rd Ed. John Wiley & Sons, 2017.
Gomes, T. C. F.; Skaf, M. S. J. Comput. Chem. 2012, 33, 1338-1346.
Liu, F.; Nielsen, A. H.; Vollertsen, J. Water 2019, 11, 526. DOI: https://doi.org/10.3390/w11030526
Lonappan, L.; Brar, S. K.; Das, R. K.; Verma, M.; Surampalli, R. Y. Environ. Int. 2016, 96, 127–138. DOI: https://doi.org/10.1016/j.envint.2016.09.014
Hobza, P. Annu. Rep. Prog. Chem., Sect. C: Phys. Chem. 2011, 107, 148-168.
Lancheros, J. C.; Madera-Parra, C. A.; Caselles-Osorio, A.; Torres-López, W. A.; Vargas-Ramírez, X. M. Ecological Engineering 2019, 135, 89–97. DOI: https://doi.org/10.1016/j.ecoleng.2019.05.007
Hu, B.; Hu, S.; Chen, Z.; Vymazal, J. J. Hazard. Mater. 2021, 409, 124524. DOI: https://doi.org/10.1016/j.jhazmat.2020.124524
Hijosa-Valsero, M.; Reyes-Contreras, C.; Domínguez, C.; Bécares, E.; Bayona, J. M. Chemosphere 2016, 145, 508–517. DOI: https://doi.org/10.1016/j.chemosphere.2015.11.090
Ávila, C.; Nivala, J.; Olsson, L.; Kassa, K.; Headley, T.; Mueller, R. A.; Bayona, J. M.; García, J. Sci. Total Environ. 2014, 494-495, 211–217. DOI: https://doi.org/10.1016/j.scitotenv.2014.06.128
Ilyas, H.; Masih, I.; van Hullebusch, E. D. Water Sci. Technol. 2021, 83, 2809–2828. https://doi.org/10.2166/wst.2021.178
Buemi, G.; Zuccarello, F. Chem. Phys. 2004, 306, 115–129. DOI: https://doir.org/10.1016/j.chemphys.2004.07.02
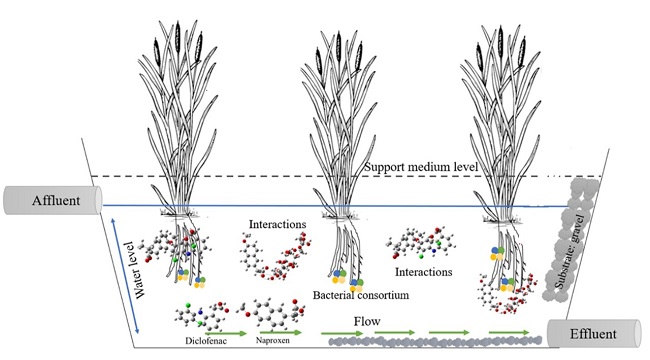
Downloads
Published
Issue
Section
License
Copyright (c) 2023 Ana Laura Zapata-Morales, Sarai Vega-Rodriguez, Ma Catalina Alfaro de la Torre, Alejandro Hernández-Morales, Socorro Leyva-Ramos, Ruth Elena Soria-Guerra

This work is licensed under a Creative Commons Attribution-NonCommercial 4.0 International License.
Authors who publish with this journal agree to the following terms:
- Authors retain copyright and grant the journal right of first publication with the work simultaneously licensed under a Creative Commons Attribution License that allows others to share the work with an acknowledgement of the work's authorship and initial publication in this journal.
- Authors are able to enter into separate, additional contractual arrangements for the non-exclusive distribution of the journal's published version of the work (e.g., post it to an institutional repository or publish it in a book), with an acknowledgement of its initial publication in this journal.
