Aluminum Clusters (N=2-6) on CTF-0 Monolayer for Adsorption of Atrazine: Investigated by Density Functional Theory
DOI:
https://doi.org/10.29356/jmcs.v66i3.1760Keywords:
CFT-0, aluminium, adsorption, atrazine, DFTAbstract
Abstract. We present a theoretical investigation of the structural characteristics and stabilities of neutral and positively charged aluminum clusters Aln n= 2-6 on covalent triazine frameworks (CTF-0). We found that clusters are adsorbed on the CTF-0 with adsorption energies of 26.32 – 91.53 kcal/mol. All calculations showed that the interaction between the aluminum cluster and CTF-0 is strong and prefers to adsorb in the central cavity of the monolayer with Al-C 2.03-2.92 Å and Al-N 1.89-2.12 Å bond formation. Next, we calculated the adsorption of an atrazine molecule on the [AlnCTF-0] n=2-6 systems. It is found that an atrazine molecule is physically adsorbed only on three systems, [AlnCTF-0] n=3-4,6 with adsorption energies in the range of 30.68 to 61.08 kcal/mol. The NBO analysis reveals that aluminum atoms accept electron density from nitrogen of atrazine molecule, but they also return electron density to the atrazine molecule. Although this result suggests that the [AlnCTF-0] n = 3-4,6 systems can be used as a promising candidate to remove the atrazine molecule.
Resumen. Presentamos una investigación teórica de las características estructurales y las estabilidades de los cúmulos de aluminio cargados positivamente y neutros Aln n = 2-6 en el fragmento de triazina covalente (CTF-0). Encontramos que los cúmulos se adsorben en el CTF-0 con energías de adsorción de 26.32 a 91.53 kcal/mol. Todos los cálculos mostraron que la interacción entre el cumulo de aluminio y CTF-0 es fuerte y prefiere adsorberse en la cavidad central de la monocapa con formación de enlaces Al-C 2.03-2.92 Å y Al-N 1.89-2.12 Å. Seguido, calculamos la adsorción de una molécula de atrazina en los sistemas [AlnCTF-0] n=2-6. Se encuentra que una molécula de atrazina se adsorbe físicamente sólo en tres sistemas, [AlnCTF-0] n=3-4,6 con energías de adsorción en el rango de 30.68 a 61.08 kcal/mol. El análisis NBO revela que los átomos de aluminio aceptan la densidad de electrones del nitrógeno de la molécula de atrazina, pero también devuelven densidad de electrones a la molécula de atrazina. Estos resultados sugieren que los sistemas [AlnCTF-0] n = 3-4,6 pueden usarse como candidatos prometedores para remover la molécula de atrazina.
Downloads
References
Kyle, D. J. Photochem. Photobiol. 1985, 41, 107-116. DOI: https://doi.org/10.1111/j.1751-1097.1985.tb03456.x
Hirschberg, J.; Mcintosh, L. Science. 1983, 222, 1346-1349. DOI: https://doi.org/10.1126/science.222.4630.1346
Silva, C. R.; Gomes, T. F.; Andrade, G. C. R. M.; Monteiro, S. H.; Dias, A. C. R.; Zagatto, E. A. G.; Tornisielo, V. L. J. Agr. Food Chem. 2013, 61, 2358-2363. DOI: https://doi.org/10.1021/jf304742h
Boyd, R. A. Sci. Total Environ. 2000, 248, 241-253. DOI: https://doi.org/10.1016/S0048-9697(99)00546-X
Thomas, D. H.; Beck-Westermeyer, M.; Hage, D. S. Anal. Chem. 1994, 66, 3823-3829. DOI: https://doi.org/10.1021/ac00093a044
Rostami, S.; Jafari, S.; Moeini, Z.; Jaskulak, M.; Keshtgar, L.; Badeenezhad, A.; Azhdarpoor, A.; Rostami, M.; Zorena, K.; Dehghani, M. Environ. Technol. Innov. 2021, 24, 102019. DOI: https://doi.org/10.1016/j.eti.2021.102019
Yildirim, M.; Kaya, V.; Yildiz, M.; Demirpence, O.; Gunduz, S.; Dilli, U. D. Asian Pac. J. Cancer. Prev. 2014, 15, 2821–2823. DOI: https://doi.org/10.7314/apjcp.2014.15.6.2821
P., B.; H. O., A.; C., B.; J. S., M. Eur. J. Cancer Prev. 2013, 22, 169–80. DOI: https://doi.org/10.1097/cej.0b013e32835849ca
Hua, W.; Bennett, E. R.; Letcher, R. J. Water Res. 2006, 40, 2259-2266. DOI: https://doi.org/10.1016/j.watres.2006.04.033
Zhang, J.; Liang, S.; Wang, X.; Lu, Z.; Sun, P.; Zhang, H.; Sun, F. Biomed. Res. Int. 2019, 4756579-4756579. DOI: https://doi.org/10.1155/2019/4756579
Macías-Flores, A.; Tafoya-Garnica, A.; Ruiz-Ordaz, N.; Salmerón-Alcocer, A.; Juárez-Ramírez, C.; Ahuatzi-Chacón, D.; Mondragón-Parada, M.; Galíndez-Mayer, J. World J. Microbiol. Biotechnol. 2009, 25, 2195. DOI: https://doi.org/10.1007/s11274-009-0125-0
Tao, Y.; Hu, S.; Han, S.; Shi, H.; Yang, Y.; Li, H.; Jiao, Y.; Zhang, Q.; Akindolie, M. S.; Ji, M.; Chen, Z.; Zhang, Y. Sci. Total Environ. 2019, 682, 59-69. DOI: https://doi.org/10.1016/j.scitotenv.2019.05.134
Akpinar, I.; Drout, R. J.; Islamoglu, T.; Kato, S.; Lyu, J.; Farha, O. K. ACS Appl. Mater. Interfaces 2019, 11, 6097-6103. DOI: https://doi.org/10.1021/acsami.8b20355
Bayati, M.; Numaan, M.; Kadhem, A.; Salahshoor, Z.; Qasim, S.; Deng, H.; Lin, J.; Yan, Z.; Lin, C.-H.; Fidalgo de Cortalezzi, M. J. Environ. Chem. Eng. 2020, 8, 104407. DOI: https://doi.org/10.1016/j.jece.2020.104407
Akpinar, I.; Yazaydin, A. O. J. Chem. Eng. Data 2018, 63, 2368-2375. DOI: https://doi.org/10.1021/acs.jced.7b00930
Zhou, Y.; Zhang, F.; Tang, L.; Zhang, J.; Zeng, G.; Luo, L.; Liu, Y.; Wang, P.; Peng, B.; Liu, X. Sci. Rep. 2017, 7, 43831. DOI: https://doi.org/10.1038/srep43831
Moreno-Rodríguez, D.; Jankovič, J.; Scholtzová, E.; Tunega, D. Minerals. 2021, 11, 554. DOI: https://doi.org/10.3390/min11060554
Meng, Z.; Robert Carper, W. J. Mol. Struct. (Theochem) 2000, 531, 89-98. DOI: https://doi.org/10.1016/S0166-1280(00)00428-0
Kumar, V.; Kumar, V.; Upadhyay, N.; Sharma, S. Biotech. 2015, 5, 791-798. DOI: https://doi.org/10.1007/s13205-015-0281-x
Belzunces, B.; Hoyau, S.; Benoit, M.; Tarrat, N.; Bessac, F. J. Comput. Chem. 2017, 38, 133-143. DOI: https://doi.org/10.1002/jcc.24530
Hao, L.; Ning, J.; Luo, B.; Wang, B.; Zhang, Y.; Tang, Z.; Yang, J.; Thomas, A.; Zhi, L. J. Am. Chem. Soc. 2015, 137, 219-225. DOI: https://doi.org/10.1021/ja508693y
Katekomol, P.; Roeser, J.; Bojdys, M.; Weber, J.; Thomas, A. Chem. Mater. 2013, 25, 1542-1548. DOI: https://doi.org/10.1021/cm303751n
Jiang, X.; Wang, P.; Zhao, J. J. Mater. Chem. A 2015, 3, 7750-7758. DOI: https://doi.org/10.1039/C4TA03438D
Dapprich, S.; Komáromi, I.; Byun, K. S.; Morokuma, K.; Frisch, M. J. J. Mol. Struct. (Theochem) 1999, 462, 1-21. DOI: https://doi.org/10.1016/S0166-1280(98)00475-8
Adamo, C.; Barone, V. J. Chem. Phys. 1999, 110, 6158-6170. DOI: https://doi.org/10.1063/1.478522
Perdew, J. P.; Burke, K.; Ernzerhof, M. Phys. Rev. Lett. 1996, 77, 3865-3868. DOI: https://doi.org/10.1103/PhysRevLett.77.3865
Perdew, J. P.; Burke, K.; Ernzerhof, M. Phys. Rev. Lett. 1997, 78, 1396-1396. DOI: https://doi.org/10.1103/PhysRevLett.78.1396
Weigend, F.; Ahlrichs, R. Phys. Chem. Chem. Phys. 2005, 7, 3297-3305. DOI: https://doi.org/10.1039/B508541A
Binkley, J. S.; Pople, J. A.; Hehre, W. J. J. Am. Chem. Soc. 1980, 102, 939-47. DOI: https://doi.org/10.1021/ja00523a008
Krishnan, R.; Binkley, J. S.; Seeger, R.; Pople, J. A. J. Phys. Chem. 1980, 72, 650-654. DOI: https://doi.org/10.1063/1.438955
Glendening, E. D.; Landis, C. R.; Weinhold, F. J. Comp. Chem. 2013, 34, 1429-1437. DOI: https://doi.org/10.1002/jcc.23266
Stefan, G.; Jens, A.; Stephan, E.; Helge, K. J. Chem. Phys. 2010, 132, 154104. DOI: https://doi.org/10.1063/1.3382344
Chung, L. W.; Sameera, W. M. C.; Ramozzi, R.; Page, A. J.; Hatanaka, M.; Petrova, G. P.; Harris, T. V.; Li, X.; Ke, Z.; Liu, F.; Li, H.-B.; Ding, L.; Morokuma, K. Chem. Rev. 2015, 115 , 5678-5796. DOI: https://doi.org/10.1021/cr5004419
Wang, Y.; Li, J.; Yang, Q.; Zhong, C. ACS Appl. Mater. Interfaces 2016, 8, 8694-8701. DOI: https://doi.org/10.1021/acsami.6b00657
Kiohara, V. O.; Carvalho, E. F. V.; Paschoal, C. W. A.; Machado, F. B. C.; Roberto-Neto, O. Chem. Phys. Lett. 2013, 568-569, 42-48. DOI: https://doi.org/10.1016/j.cplett.2013.03.005
Maatallah, M.; Guo, M.; Cherqaoui, D.; Jarid, A.; Liebman, J. F. Int. J. Hydrog. Energy. 2013, 38, 5758-5767. DOI: https://doi.org/10.1016/j.ijhydene.2013.03.015
Jones, R. O. Phys. Rev. Lett. 1991, 67, 224-227. DOI: https://link.aps.org/doi/10.1103/PhysRevLett.67.224
Kumar, D.; Pal, S.; Krishnamurty, S. Phys. Chem. Chem. Phys. 2016, 18, 27721-27727. DOI: https://doi.org/10.1039/C6CP03342C
Luo, J.; Xue, Z. Q.; Liu, W. M.; Wu, J. L.; Yang, Z. Q. J. Phys. Chem. A 2006, 110, 12005-12009. DOI: https://doi.org/10.1021/jp063669m
Hicken, A.; White, A. J. P.; Crimmin, M. R. Inorg. Chem. 2017, 56, 8669-8682. DOI: https://doi.org/10.1021/acs.inorgchem.7b00182
Miertuš, S.; Scrocco, E.; Tomasi, J. Chem. Phys. 1981, 55, 117-129. DOI: https://doi.org/10.1016/0301-0104(81)85090-2
Sahithi, A.; Sumithra, K. RSC Adv. 2020, 10, 42318-42326. DOI: https://doi.org/10.1039/D0RA06760A
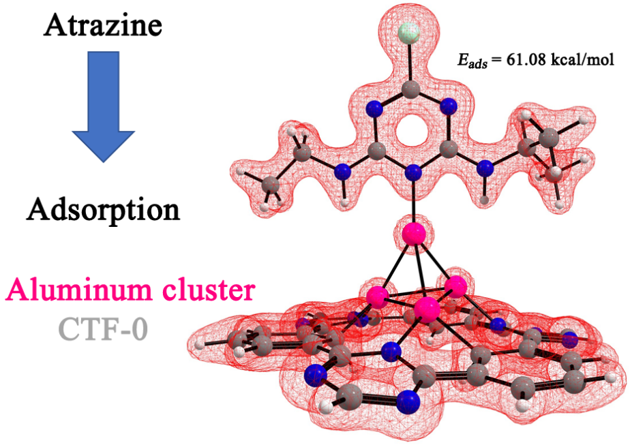
Downloads
Published
Issue
Section
License
Copyright (c) 2022 Marisol Ibarra Rodríguez

This work is licensed under a Creative Commons Attribution-NonCommercial 4.0 International License.
Authors who publish with this journal agree to the following terms:
- Authors retain copyright and grant the journal right of first publication with the work simultaneously licensed under a Creative Commons Attribution License that allows others to share the work with an acknowledgement of the work's authorship and initial publication in this journal.
- Authors are able to enter into separate, additional contractual arrangements for the non-exclusive distribution of the journal's published version of the work (e.g., post it to an institutional repository or publish it in a book), with an acknowledgement of its initial publication in this journal.
