Impurity Defect Induced Ferromagnetism Investigation of SiO2-Supported NiO Particles
DOI:
https://doi.org/10.29356/jmcs.v66i1.1623Keywords:
Transition metal oxides, co-precipitation, photoluminescence, magnetismAbstract
Abstract. This study examines amorphous SiO2-supported NiO particles by nickel concentration and calcination temperature arrangement to determine photoluminescence emission peaks and magnetic properties. Conventional co-precipitation with thermal calcination was used to produce NiO nanoparticles. Cubic NiO crystallization with single phase was improved by doubling the nickel concentration by calcination at 500 ºC and 600 ºC. Average crystalline size of 72 nm was obtained in the samples where double nickel concentration with calcination temperature at 600 ºC. Granular forms have been observed in all samples, and nickel clusters were shown in the samples where the nickel concentration is twice as high. Green band emission intensity increases with improved NiO crystallinity due to surface oxygen vacancies at 505 nm. It is interesting to observe ferrimagnetism for SiO2-supported NiO particles calcined at 500 ºC. From these results, optimal synthesis procedure and reduction in nucleation growth of NiO nanoparticles was achieved by double nickel concentration with calcination temperature at 600 ºC.
Resumen. Este estudio examina partículas de NiO soportadas en SiO2. El estudio comprende la variación de la concentración de níquel y la temperatura de calcinación para determinar los picos de emisión de fotoluminiscencia y las propiedades magnéticas. Se utilizó la coprecipitación convencional con calcinación para producir nanopartículas de NiO. Se mejoró la cristalización cúbica de NiO con fase única al duplicar la concentración de níquel y calcinación a 500 ºC y 600 ºC. Se obtuvo un tamaño cristalino promedio de 72 nm en las muestras donde se duplicó la concentración de níquel con temperatura de calcinación a 600 ºC. Se observaron formas granulares en todas las muestras, y se encontraron agregados de níquel en las muestras donde la concentración de níquel fue el doble. La intensidad de la banda de emisión aumenta con la cristalinidad de NiO debido a las vacantes de oxígeno en la superficie. Es interesante observar el ferrimagnetismo de las partículas de NiO soportadas en SiO2 calcinadas a 500 ºC. A partir de estos resultados, se logró un procedimiento de síntesis óptimo y la reducción del crecimiento de nucleación de nanopartículas de NiO mediante una concentración doble de níquel con una temperatura de calcinación de 600 ºC.
Downloads
References
Orbeck, J.K.H.; Hamers, R.J. J. Vac. Sci. Technol. A. 2020, 38, 031001.
Issa, B; Obaidat, I.M.; Albiss, B.A.; Haik, Y. Int J. Mol. Sci. 2013, 14, 21266-21305.
Poizot, P; Laruelle, S; Grugeon, S.; Dupont, L.; Tarascon, J.M. Nature. 2000, 407, 496–499.
Arif, M.; Sanger, A; Mohd. Shkir, Singh, A.; Katiyar, R.S. Physica B: Condensed Matter. 2019, 552, 88-95.
Gandhi, A.C.; Wu, S.Y. AIP Advances. 2019, 10, 015211-015221.
Adhikary, J.; Chakraborty, P.; Das, B.; Datta, B.; Dash, S. K.; Roy, Chen, S. J.; Chattopadhyay, T. RSC Adv. 2015, 5, 35917.
Wang, C.; Tian, F.; Zhang, X. Mater. Res. Express. 2020, 7, 106403.
Sowe, M.S.; Masruri; Ulfa, S.M. IOP Conf. Ser.: Mater. Sci. Eng. 2019, 546, 072007-072213.
Husain, H.; Hariyanto, B.; Sulthonul, M.; Klysubun, W.; Darminto, D.; Pratapa, S. Mater. Res. Express, 2019, 6, 086117
Kolthoff, M. J. Phys. Chem. 1932, 36, 860–881.
Nakouzi, E.; Steinbock, O. Science Advances. 2016, 2, e1601144.
Rabiei, M.; Palevicius, A.; Monshi, A.; Nasiri, S.; Vilkauskas, A.; Janusas, G. Nanomaterials. 2020, 10, 1627-1638.
Terohid, S. A. A.; Heidari, S.; Jafari, A.; Asgary, S. Applied Physics A. 2018, 124, 567-576.
Tinio, J.V.G.; Simfroso, K.T.; Peguit, A.D.M.V.; Candidato, Jr. R.T. J. Nanotechnol. 2015, 686021, 7.
Holder, C.F.; Schaak, R.E. ACS Nano. 2019, 13, 7359?7365.
Gandhi, A.C.; Wu, S.Y. Nanomaterials (Basel). 2017, 7, 231.
Ishizuka, H.; Nagaosa, N. New J. Phys. 2018, 20, 123027.
Park, H.; Ahn, C.; Ki Lee, H.; Kim, H.; Jin, H. Lee, Seo, S.; Yu, J.; Han, S. Phys. Rev. B. 2008, 77, 134103.
Musevi, S.J.; Aslani, A.; Motahari, H. S. J. Saudi Chem. Soc. 2016, 20, 245–252.
Patel, K. N. et. al., Mater. Res. Express. 2017, 4, 105027.
Kumar, V.P.; Ahamed, A.J.; Karthikeyan, M. SN Appl. Sci. 2019, 1, 1083.
Watthage, S. C.; Song, Z.; Shrestha, N.; Phillips, A. B.; Liyanage, G. K.; Roland, P. J. ; Ellingson, R. J. ; Heben, M. J. ACS Appl. Mater. Interfaces. 2017, 9, 2334?2341.
Sarf, F.; A??kuzun, E.; Yakar, E. Int. J. Adv. Eng. Pure Sci. 2021, 33, 52-57.
Mochizuki, S.; Saito, T. Physica B. 2009, 404, 4850–4853.
Nadeem, K.; Ullah, A; Mushtaq, M.; Kamran, M.; Hussain, S.S.; Mumtaz, M. J. Magn. Magn. Mater. 2016, 417, 6–10.
Ghalmi, Y.; Habelhames, Y.; Sayah, A.; Bahloul, A.; Nessark, B.; Shalabi, M.; Nunzi, J. M. Ionics. 2019, 25, 6025–6033.
Oh, T.; Choi, C. J. Korean Phys. Soc. 2010, 56, 1150-1155.
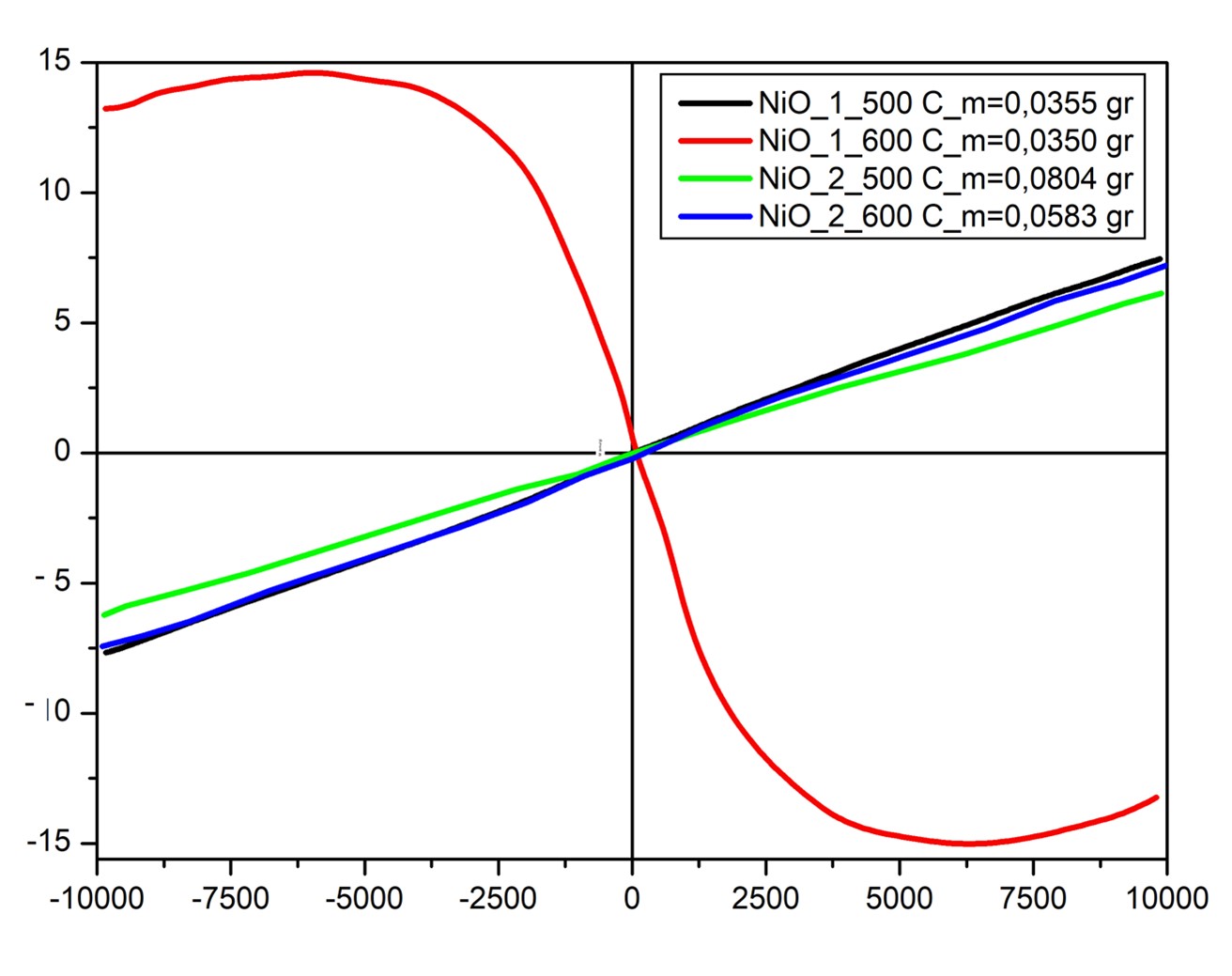
Downloads
Published
Issue
Section
License
Authors who publish with this journal agree to the following terms:
- Authors retain copyright and grant the journal right of first publication with the work simultaneously licensed under a Creative Commons Attribution License that allows others to share the work with an acknowledgement of the work's authorship and initial publication in this journal.
- Authors are able to enter into separate, additional contractual arrangements for the non-exclusive distribution of the journal's published version of the work (e.g., post it to an institutional repository or publish it in a book), with an acknowledgement of its initial publication in this journal.
